Table of Contents
Introduction
There are many possible causes of concrete deterioration. Concrete is most often damaged by corrosion of rebar due to carbonation of hardened cement paste or by chloride attack in wet conditions.
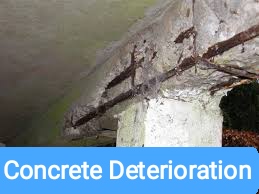
Chemical damage is caused by various chemical reactions, aggressive chemical species (chloride, sulfate, magnesium ions) present in groundwater and seawater, or the formation of expansive products caused by microorganisms such as bacteria, fungi, etc.
Other detrimental processes also include calcium leaching through water infiltration and various physical phenomena that initiate crack formation and propagation.
All these adverse processes and harmful substances affect the mechanical strength of concrete and its durability. Water is probably the most damaging to concrete structures and components. In fact, water, as a reagent, is often directly involved in chemical reactions and is always required as a solvent or reaction medium to enable transport and reaction of solutes. Without water, many detrimental reactions do not occur or occur so slowly that their detrimental consequences are negligible during the planned service life of the structure.
Dry concrete has a much longer service life than wet concrete in contact with circulating water. Therefore, concrete should first of all be protected from water ingress, if possible.
Causes of Concrete Deterioration
1. Corrosion of Rebar
The expansion of corrosion products in carbon steel reinforcement structures can induce internal mechanical stresses that cause cracks and destroy concrete structures.
Improperly installed rebar or poor concrete coverage on surfaces exposed to the elements can result in oxide drift and spalling during the life of the structure.
Corrosion of rebar separates flat pieces of concrete from the concrete mass.
Rebar is required to support the tensile forces experienced by concrete in most civil engineering structures, and stainless steel is too expensive to replace carbon steel.
Galvanizing or epoxy coating can improve the corrosion resistance of rebar, but it also has other drawbacks such as surface adhesion to concrete , which can form cathodic and anodic zones that promote galvanic corrosion if the protective coating is locally punctured or damaged , and their high cost.
2. Formation of the Swelling Stage of Concrete
As a hard rock, concrete can withstand high compressive stresses, but not tensile stresses. As a result, concrete is easily damaged when expansion stages form in its mass.
The most ubiquitous and well-known expansion phase is probably iron oxide produced by the oxidation of carbon steel rebars embedded in concrete.
Corrosion products form around rebar in carbonate concrete directly exposed to atmospheric oxygen when cracks form. Corrosion damage to rebar is clearly visible to the naked eye and easy to diagnose. Other detrimental expansive chemical reactions that are more difficult to characterize and identify can occur in concrete.
First of all, they can be distinguished according to their place of occurrence in concrete.
in aggregate or in hardened cement paste.
3. Chemical Damages
Concrete performs well when exposed to a variety of atmospheric conditions such as water, soil, and many other chemical stresses. However, some chemical environments can degrade even good quality concrete. Concrete is seldom, if ever, attacked by solid dry chemicals. Aggressive chemicals must be dissolved and above certain minimum concentrations to cause significant attack on concrete.
4. Effects of Bacterial Activity
The bacteria themselves do not noticeably affect concrete. However, sulphate-reducing bacteria tend to produce hydrogen sulfide (H2S) in untreated wastewater, which is then used by atmospheric oxygen and aerobic bacteria present in biofilms.
Concrete floors over pyrite-bearing subsoil (iron disulfide) are also at risk. As a precaution, wastewater can be pretreated to raise the pH or oxidize or precipitate sulfides to minimize the activity of sulfide-reducing bacteria.
4. Freeze Thaw Cycles
In winter or in cold climates, ice crystallization within the pores of concrete is also a physical mechanism responsible for volumetric expansion of high tensile strength materials within concrete when the temperature drops below 0 degrees Celsius.
Cracks occur when the tensile strength of concrete is exceeded. The addition of air entraining agents when mixing ready-mixed concrete results in the formation of small air bubbles in the ready-mixed concrete suspension. This creates numerous tiny air-filled micro voids in the hardened concrete. It acts as a void volume reserve to accommodate the volumetric expansion of the ice and to delay the moment of tensile stress. Air entrapment improves workability during concrete placement and increases post-cure durability, especially in climates prone to freeze-thaw cycles.
5. Thermal Damages
Due to its low thermal conductivity, concrete layers are often used for fire protection in steel structures.
Up to about 300 °C, concrete experiences normal thermal expansion. Above this temperature, shrinkage occurs due to moisture loss. However, the aggregate continues to expand, causing internal stress. The main structural changes up to about 500 °C are carbonation and pore coarsening. Quartz expands rapidly due to phase transition at 573°C, and calcite begins to contract due to decomposition at 900°C. Cement hydrate decomposes to calcium oxide at 450-550 °C. Calcium carbonate decomposes at about 600°C.
As the structure cools, rehydration of the calcium oxide causes expansion, which can damage materials that have survived the fire without falling apart indicating a high degree of carbonation due to reabsorbed carbon dioxide.
Explosive spalling of concrete can occur when concrete is exposed to very high temperatures very quickly. In a very hot, very fast fire, the water in the concrete boils before it evaporates. Steam inside concrete exerts an expansion pressure that can cause spalling and violent ejection.
6. Mechanical Damages
Overloads, shocks and vibrations can cause mechanical stresses and deformations in concrete structures, leading to mechanical deterioration of concrete.
In addition to the long-term drying shrinkage of concrete, prestressed and post-tensioned engineering structures can also be exposed to slow concrete creep and deformation.
7. Radiation Damage
Exposure of concrete structures to neutron and gamma rays in nuclear power plants and high-flux materials testing reactors can lead to radiation hazards in concrete structural components. Paramagnetic defects and optical centers are readily formed, but require a very high flux is required.
Very high neutron fluence are known to amorphize some of the quartz present in some concrete aggregates. This amorphization process is also known as metamitization. Metamictic quartz with a disordered lattice structure is susceptible to alkali-silica reactions, which can lead to detrimental chemical expansion in the concrete of nuclear containment structures.