What Is An Earthquake Resistant Building?
Whenever an earthquake strikes it comes without giving a single hint of it. Everything about it is unpredictable. We don’t know when it is coming, how it is coming, how strong it is, how long will it last; everything is known after it happens. Hence, it is to be noted that constructing a structure which is 100% earthquake proof is probably impossible. We can only go on increasing the resistance of a structure against earthquake and that too by considering the past experiences. Many engineers and architects now believe it’s possible to build an earthquake-proof building — one that would ride the waves of the most fearsome temblor and remain as good as new once the shaking had stopped. The cost of such a building, however, would be staggering. Instead, construction experts strive for something slightly less ambitious — earthquake-resistant buildings, which are designed to prevent total collapse and preserve life, as well as construction budgets.
During an earthquake seismic loads act on a building as lateral loads. So basically an earthquake resistant building is a type of structure which provides resistance to additional lateral loads with sudden effect in addition to the vertical live loads and dead loads plus the lateral wind loads. They are designed by considering certain requirements. These requirements take into consideration the characteristics and probability of occurrence of earthquakes, the characteristics of the structure and the foundation, and the amount of damage that is considered tolerable.
Seismic Loads
Seismic loads are of kinematic origin. They owe their existence to vibrations caused in structures by the movement of the earth’s surface during an earthquake. Seismic loads are random in nature, though they are usually regarded as deterministic in practical calculations to simplify the design model. The study of the generation, propagation, sources recording of elastic waves in the earth which leads to the generation of seismic loads is called engineering seismology.
Structures on earth are generally subjected to two types of loads, statics loads and dynamic loads. Static loads are constant with respect to time while dynamic loads are time dependent. While dealing with earthquake static loads are not much important. In terms of confidence in their values dynamic loads may be classified as:-
1) Deterministic loads- They are always prescribed and they are functions of time.
2) Stochastic or random loads-their determination implies a certain probabilistic element.
Seismic loads result directly from the distortions induced in the structure by the motion of the ground on which it rests. Base motion is characterized by displacements, velocities, and accelerations that are erratic in direction, magnitude, duration, and sequence. Earthquake loads are inertia forces related to the mass, stiffness, and energy-absorbing (e.g., damping and ductility) characteristics of the structure.
Seismic Design Concept
An effective seismic design generally includes:
1. Selecting an overall structural concept including layout of a lateral force resisting system that is appropriate to the anticipated level of ground shaking. This includes providing a redundant and continuous load path to ensure that a building responds as a unit when subjected to ground motion.
2. Determining code-prescribed forces and deformations generated by the ground motion, and distributing the forces vertically to the lateral-force-resisting system. The structural system, configuration, and site characteristics are all considered when determining these forces.
3. Analysis of the building for the combined effects of gravity and seismic loads to verify that adequate vertical and lateral strength and stiffness are achieved to satisfy the structural performance and acceptable deformation levels prescribed in the governing building code.
4. Providing details to assure that the structure has sufficient inelastic deform-ability to undergo fairly large deformations when subjected to a major earthquake. Appropriately detailed members possess the necessary characteristics to dissipate energy by inelastic deformations.
BEHAVIOR OF RCC BUILDINGS UNDER SEISMIC LOADS
During an earthquake, ground motions occur in a random manner, both horizontally and vertically, in all directions, radially coming from the epicenter of the earthquake. The ground accelerations cause the structures to vibrate and induce inertial forces on them. The resultant inertial force at any floor level on the mass at the floor level and also its height above the foundation. The inertial forces usually follow a parabolic distribution in a regular multi-storey building, with maximum loads on the top floor levels. Torsional effects are also encountered in buildings with asymmetry in plan or elevation.
Diagram below shows the seismic load path through a building
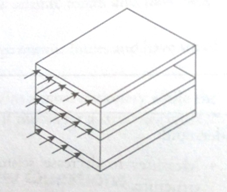
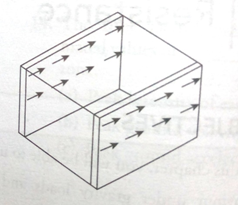
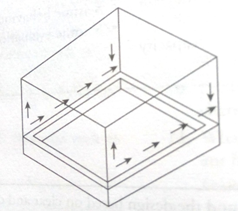
Seismic Design Philosophy
The philosophy of seismic design can be summarized as:The design philosophy adopted in the code is to ensure that the structures possess at least a minimum strength to (a) Resist minor earthquake (< DBE), which may occur frequently, without damage. (b) Resist moderate earthquake (DBE) without significant structural damage through some non-structural damage. (c) resist major earthquake (MCE) without collapse.
“Design Basis Earthquake (DBE) is defined as the maximum earthquake that reasonably can be expected to experience at the site once during lifetime of the structure. The earthquake corresponding to the ultimate safety requirements is often called as Maximum Considered Earthquake (MCE). Generally, the DBE is half of MCE.”
- The basic criteria of earthquake resistant design should be based on lateral strength as well as deformability and ductility capacity of structure with limited damage, but no collapse.
- The design lateral forces specified in the code shall be considered in each of the orthogonal directions of the structure.
- Earthquake generated vertical inertia forces are to be considered in design unless it is not significant. Vertical acceleration should be considered in structures with large spans, those in which stability is a criterion for design, or for overall stability analysis of structures. Reduction gravity forces due to vertical components of ground motions can be particularly detrimental in case of pre-stressed horizontal members and of cantilevered members. Hence, special attention should be paid to the effect of vertical component of the ground motion on pre-stressed or cantilevered beams, girders and slab. Where both horizontal and vertical seismic forces are taken into account, load combination specified in the code shall be considered.
- The response of a structure to ground vibrations is a function of the nature of foundation soil; materials, form, size and mode of construction of structures; and the duration and characteristics of ground motion. The code specifies design forces for structures standing on rock or firm soils, which do not liquefy or slide due to loss of strength during ground vibrations.
Requirements Of An Earthquake Resistant Buildings
The criteria adopted by codes for fixing the level of the design seismic loading are generally as follows:
- Structures should be able to resist minor earthquakes without damage;
- Structures should be able to resist moderate earthquakes without significant structural damage, but with some non-structural damage; and
- Structures should be able to resist major earthquakes without collapse, but with some structural and non-structural damage.
WAYS TO MINIMIZE THE EFFECTS OF EARTHQUAKE ON BUILDINGS
A good lateral load resisting structure can ensure inelastic behaviour by having greater redundancy, thereby having larger ductility and damping.
1) Special Moment Resisting Frames (SMRF)
Special Moment-resisting frame is a rectilinear assemblage of beams and columns, with the beams rigidly connected to the columns. Resistance to lateral forces is provided primarily by rigid frame action – that is, by the development of bending moment and shear force in the frame members and joints.
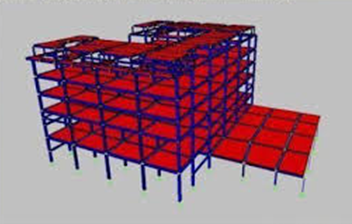
2) Shear Walls
Shearwall is a structural member used to resist lateral forces i.e. parallel to the plane of the wall. For slender walls where the bending deformation is more. Shearwall resists the loads due to Cantilever Action. In other words, Shearwalls are vertical elements of the horizontal force resisting system.
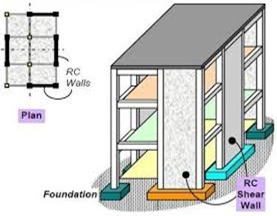
3) Braced Frames
A braced frame is a structural system designed to resist wind and earthquake forces. Members in a braced frame are not allowed to sway laterally (which can be done using shear wall or a diagonal steel sections, similar to a truss).

4) Shear Wall- Frame Interactive System (Dual Structural System)
Shear walls and frames in combination normally provide the required stiffness and strength to withstand lateral loads effectively in high rise buildings. In certain cases the walls are much stiffer than the frames and thus take most of the lateral load. For this reason, the participation of the frame in resisting lateral load is often ignored. This may not always be a conservative procedure. With the construction of increasing tall building allowing for the effect of frames and walls becomes more important. When the building is very tall, the shear wall flexural deformation become very pronounced and hence induces deformation in the frame which must be allowed for in the analysis.
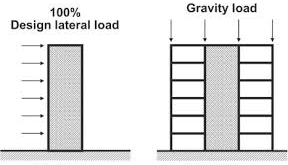
5) Coupled Shear Wall System
When two or more shear walls are connected by a system of beams or slabs, total stiffness exceeds the summation of individual stiffness. This is because the connecting beam restrains individual cantilever action. Shear walls resist lateral forces up to 30–40 storeys. Walls with openings present a complex problem to the analyst.
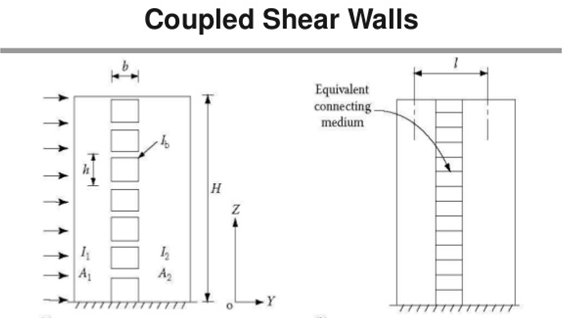
6) Frame-Tube System
Maximum Efficiency of the entire structure for lateral strength and stiffness can be achieved only by making all column elements connected to each other in such a way that the entire building acts as a hollow tube or rigid box cantilevering out of the ground. Such a system is called Framed Tube System. This system is efficient for building high rise structures.
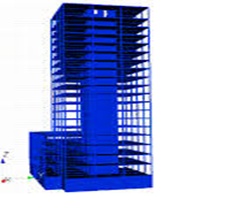
7) Outrigger Braced Shear Wall System
Nowadays, in modern tall buildings, lateral loads induced by wind or earthquake forces are often resisted by a system of multi-outriggers. An outrigger is a stiff beam that connects the shear walls to exterior columns. When the structure is subjected to lateral forces, the outrigger and the columns resist the rotation of the core and thus significantly reduce the lateral deflection and base moment, which would have arisen in a free core. During the last three decades, numerous studies have been carried out on the analysis and behavior of outrigger structures. But this question is remained that how many outriggers system is needed in tall buildings.
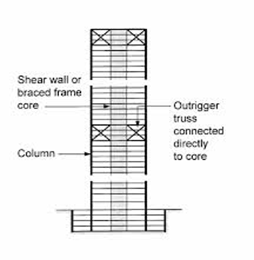
The seismic demand on the structure can be reduced by seismic modification techniques. The fundamental principle of seismic modification is to modify the response of the building to permit the dissipation of vibration energy or by isolating the building from the ground so that the ground can move below the building without/least transmission of these motions to the building.
These modification techniques are as follows:
1) Damping Devices
An approach for controlling seismic damage in buildings and improving their seismic performance is by installing Seismic Dampers in place of structural elements, such as diagonal braces. These dampers act like the hydraulic shock absorbers in cars – much of the sudden jerks are absorbed in the hydraulic fluids and only little is transmitted above to the chassis of the car. When seismic energy is transmitted through them, dampers absorb part of it, and thus damp the motion of the building.
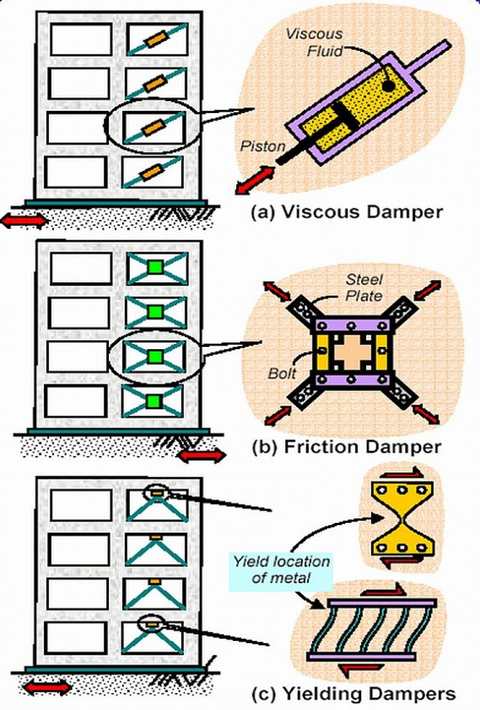
2) Base Isolation System
Base isolation is one of the most important concepts for earthquake engineering which can be defined as separating or decoupling the structure from its foundation. In other words, base isolation is a technique developed to prevent or minimize damage to buildings during an earthquake.
It might be thought that structures can be protected from the destructive forces of earthquakes by increasing the strength of the structures so that they do not collapse during such events. In other words, more rigid attachment of a building to its foundation will result in less damage in an earthquake (the principle of strengthen to resist damage). However, if the foundation is rigidly attached to the building or any other structure, all of the earthquake forces will be transferred directly and without a change in frequency to the rest of the building. Providing a base isolation device between the building and the ground can minimize the level of earthquake force transmitted to the buildings.
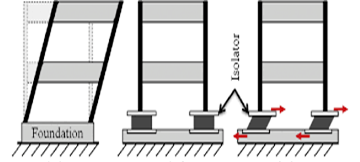
Structures that contain facilities critical to post-earthquake operations—such as hospitals, fire stations, power plants, and communication centers—must not only survive without collapse, but must also remain operational after an earthquake. Therefore, in addition to life safety, damage control is an important design consideration for structures deemed vital to post-earthquake functions.