Table of Contents
Definition
High strength concrete is a type of concrete that has a compressive strength exceeding 40 MPa. High strength concrete are significantly different from those of conventional or normal concretes.
Description
In order to produce High strength concrete, a more rigorous quality control process is required, along with careful selection and proportioning of materials.
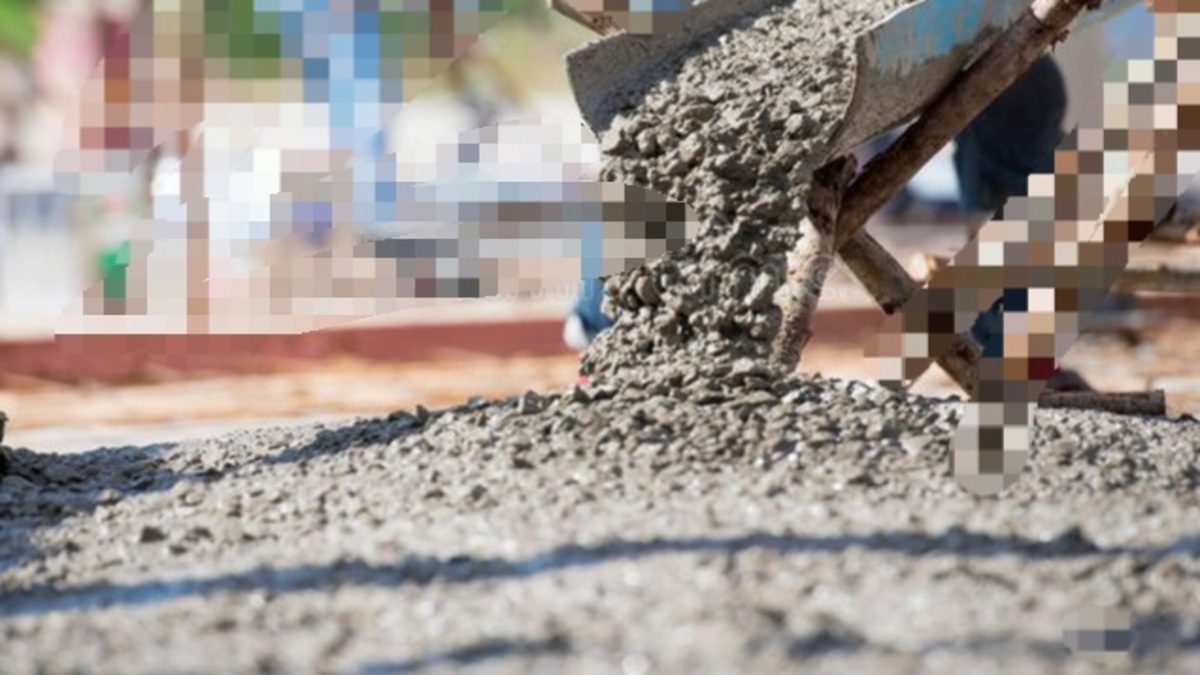
The tricalcium aluminate component kept as low as possible, ideally below 8%. Most cements used in the production of high strength concrete have a fineness in the range of 300-400 m2/kg, with the exception of high early strength cement which should have a fineness of at least 450 m2/kg. Using a smaller maximum size of coarse aggregate in High strength concrete can lead to higher strength. Fine aggregate should have a fineness modulus (FM) greater than 3.
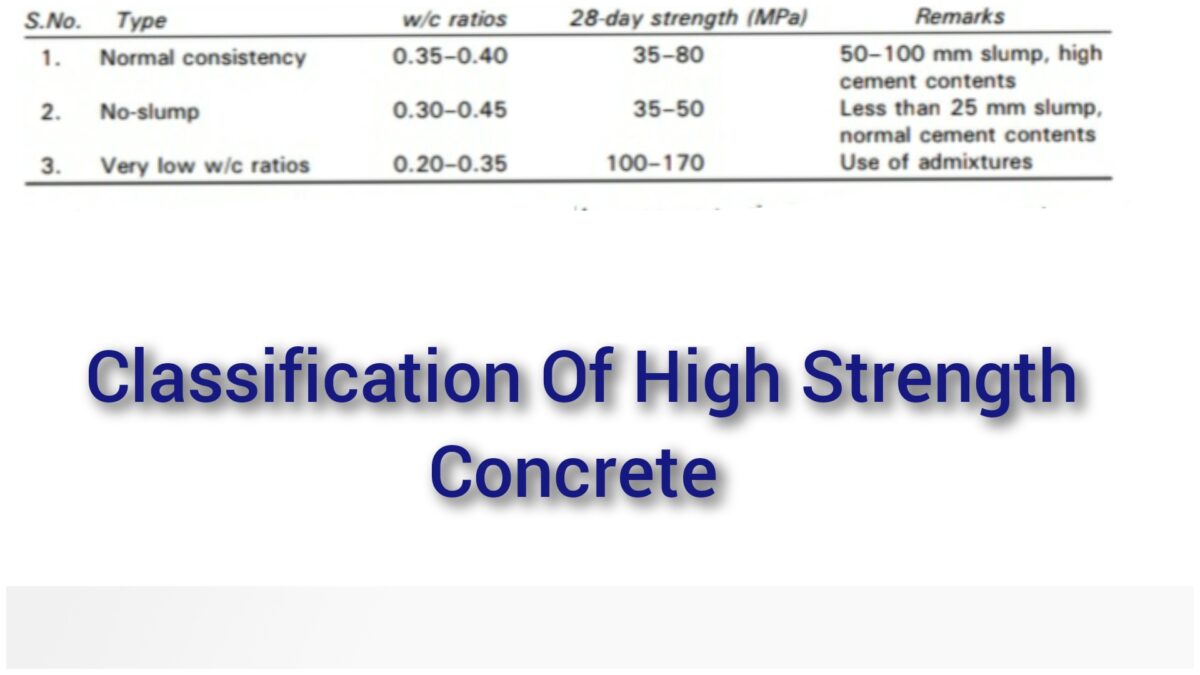
In high-strength concrete, the most important factor in determining its strength is how porous it is. The porosity of the different parts of the concrete (the aggregates, the cement paste, and the bond between them) can affect its strength.
However, it’s difficult to measure the porosity of each part separately, so we rely on empirical relations to predict strength. One well-known relation is Abram’s water/cement ratio rule, which takes into account the porosity of different parts of the concrete and other factors to estimate its strength.
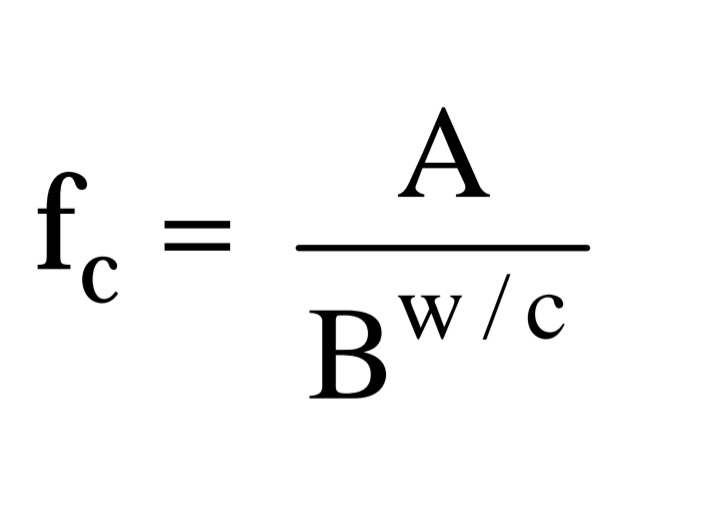
Where w/c represents the water-cement ratio of the concrete mix and A and B are empirical
constants.
In concrete production, the water-cement ratio plays a critical role in determining the porosity of both the hydrated cement paste and transition zone. Concrete producers generally observe that a lower water-cement ratio leads to achieving considerable high strength gains for very small decreases in the w/c ratio. However, decreasing water content makes fresh concrete more and more difficult to mix, place, and consolidate. Thus, to produce High-Strength Concrete (HSC), water reducing admixtures like superplasticizers are necessary to harmonize the opposing effects of w/c ratio on consistency and strength of concrete.
Reducing the maximum size of coarse aggregate can significantly increase concrete strength. This is because the transition zone is the weakest component of the HSC, and smaller aggregate size has a beneficial effect on the strength of the transition zone due to increased surface area. However, this requires a higher cement content, which can lead to inhomogeneities and micro-cracking.
To address this issue, pozzolanic materials like fly ash can be added to the mix, reducing large pores and enhancing strength. In the case of plain cement concrete (PCC), an inexpensive and effective way to achieve this is by incorporating puzzolanic materials like fly ash. Fly ash reacts with calcium hydroxide to form a reaction product that is similar in composition and properties to the principal distributions of OPC. Furthermore, the puzzolanic reaction is accompanied by a reduction in large pores, which is equally important for the enhancement of strength of the system.
When low calcium fly ash with calcium oxide content less than (6%) is used as a partial replacement of OPC, the early strength (1-and 7-day) of concrete at normal temperatures may be reduced almost in direct proportion to the amount of fly ash present in the total cementing material (cement + fly ash). However, high calcium fly ash with calcium oxide content more than 15% or ground blast furnace slag shows a significant strength within the first 7 days of hydration. Highly reactive pozzolanas like condensed silica fume and rice husk can contribute to strength even at 3 days.
The use of high-range superplasticizing admixtures is often preferred over normal water reducing admixtures when producing high-strength concrete mixes with very high consistency (200 to 250 mm slump) and strength greater than 70 MPa, while maintaining a low water-cement (w/c) ratio. While conventional superplasticizers can cause a significant increase in consistency (up to 250 mm) when added in amounts of 0.5 to 1.5% by weight of cement to a concrete mix with a 50 to 75 mm slump, the resulting high consistency concrete may revert back to its original consistency within 30 to 60 minutes. To maintain the high slump of superplasticized concrete, repeated dosages of the admixture may be necessary, although this can lead to segregation if the second or third dosage is added after slump loss. An alternative approach is to modify the admixture composition with a retarding agent to maintain the original high consistency for 3 to 6 hours.
Characteristics
1. Durability
During its initial development, the High Strength Concrete (HSC) may exhibit stickiness and stiffness, which can be attributed to the presence of a large amount of fines such as high cement content and pozzolana, a low water-cement ratio, and the use of a normal water-reducing admixture. However, advancements in superplasticizers have made it possible to achieve the desired high workability without causing any segregation, even at a lower water-cement ratio of 0.3.
2. Strength
One remarkable aspect of High-Strength Concrete (HSC) is its ability to achieve high strength quickly without requiring steam curing. Under normal curing conditions, concrete can reach 20 to 27 MPa within 24 hours, while ultra HSC can attain 42 MPa within 12 hours and 64 MPa within 24 hours.
3. Microstructure, stress-strain relation, creep and fracture
High-strength concretes (HSCs) exhibit distinctive stress-strain relation, creep, and fracture behavior due to their reduced size and number of microcracks. Unlike normal concretes, HSCs with compressive strengths between 30 and 75 MPa behave more like a homogeneous material. This is because the decrease in the amount and extent of microcracking in the transition zone makes their stress-strain curves steeper and more linear, even at higher stress-strength ratios. HSCs tend to fracture in a brittle mode and exhibit less volumetric dilation, as the amount of microcracking associated with shrinkage, short-term loading, and sustained loading is significantly lower.
4. Durability
It has been found that primarily due to low permeability, HSCs exhibit excellent durability to various physical and chemical agents that are normally responsible for concrete deterioration. Due to high cement content thermal cracking can be durability problem in structures using HSC. It has also been found that there is an expected temperature rise of 10–40°C for every 100 kg/m3 cement content.
Applications
The use of high strength concrete (HSC) and minimal steel reinforcement is considered the most cost-effective solution for columns in high-rise buildings. Research has shown that a 4.7 times increase in load carrying capacity only results in a 3.1 times increase in cost, highlighting the economic benefits of HSC in multistory buildings. Compared to steel frames, construction with concrete allows for faster building completion, as it enables construction to start before the superstructure is fully built. However, the industrial application of HSC is currently limited to structural members that are not exposed to freeze-thaw cycles.
In situations where impermeability or durability is the primary consideration, superplasticized, low water-to-cement ratio HSC containing high-quality puzzolana is an excellent choice. For instance, HSC is suitable for flooring in the chemical and food industries, as well as for bridge deck overlays that undergo severe chemical and physical degradation. The first known use of HSC was in the construction of the 53-storey Helmsley Palace Hotel in New York in 1979, where it was used to reduce the size of columns. The use of puzzolanas in concrete generates relatively less heat of hydration per unit strength, which reduces the risk of thermal cracking.
The construction of the New Tjorn Bridge in Sweden in 1981 marked the first known use of condensed silica fume in HSC, where the main consideration was heat of hydration. HSC is increasingly being selected for the construction of islands in the ocean due to its high durability in seawater environments.